The Kleiman lab
On the Interface of Materials and Biology
We use synthetic systems to separate the physical aspects from the chemical ones in plant-environment interactions.
Research Projects
1. The Effect of Leaf Surface Microstructure on Interaction with Microorganisms
We use soft lithography to replicate a tomato leaf surface microstructure, as described in the scheme:
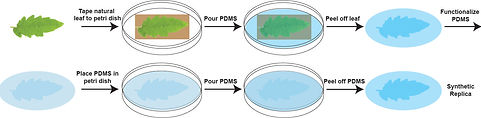
Scheme: Formation of synthetic leaf surface microstructure. We tape the natural leaf to a petri dish. We pour the liquid polymer (PDMS) and apply vacuum to assure a good cover of the microstructure. We cure the polymer at a higher temperature. We peel off the leaf from the cured polymer. At this point we have the mirror image of the leaf surface microstructure. We use this mirror image to generate the real microstructure. The same mirror image can be used repeatedly to generate identical microstructural patterns time and time again. We functionalize the mirror image to turn the polymer hydrophilic. We tape the mirror image to a petri dish and repeat the process of pouring liquid polymer, vacuum and curing. We remove the mirror image from the newly formed polymer layer, where we have the microstructure of the surface of the natural leaf replicated within the PDMS polymer.
The resulted synthetic surface resembles the original leaf surface in microstructure as can be seen in the figure below
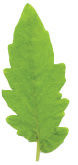

The synthetic surface will be used to study the effect of surface microstructure on leaf-environment interaction including: microorganisms, macroorganisms, liquids and air.

For example, the spread and germination of the pathogen botrytis cinerea is highly dependent on the surface microstructure.
Botrytis cinerea spread on patterned and unpatterned agar plates after 3 days incubation

Unpatterned agar surface
Patterned agar surface

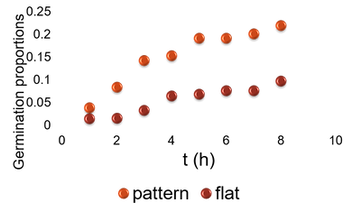
Botrytis cinerea germination proportion on patterned and unpatterned PDMS surfaces over time
Pectobacterium forms a biofilm on susceptible lily replica (right) but not on resistant one (left)

An additional example is the ability of the pathogenic bacteria Pectobacterium that causes soft rot in calla lily to form biofilm on a synthetic surface that mimics the leaf surface of the colored - sensitive lily while not forming the biofilm on a synthetic surface that mimics the leaf surface of the white - more resistant lily.
We are also testing how material's property effect these interactions. We are making our replicas from different materials and testing their physical properties

2. The Effect of Root Surface Microstructure on Interaction with Microorganisms
Similarly to the leaf, we are interested in building a synthetic root, mimicking natural root surface microstructure. Unlike the leaf, the microstructure of the root is less explored and no synthetic replica was ever made.
We developed a new method for replication of root surface structure in our lab, using polyurethane as the negative as it is liquid and could be quickly cured using UV light. Using this method we managed to replicate all relevant root features.
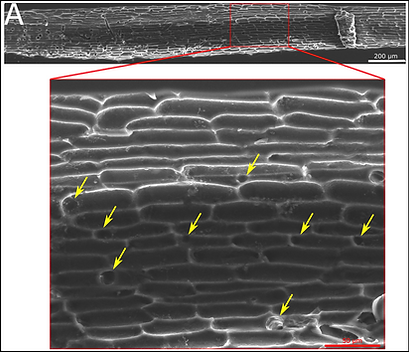
Using polyurethane for our negative replica we reproduced the root hairs as shown by the holes in the polyurethane negative, marked with yellow arrows.
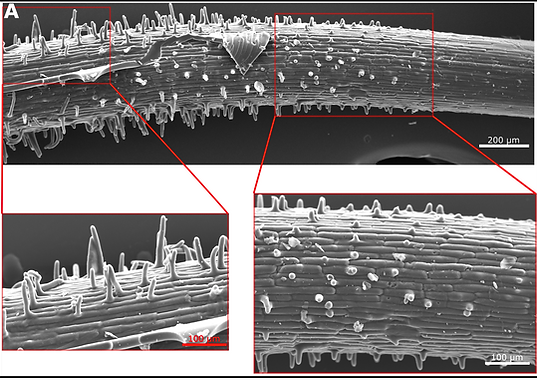
Our synthetic root replica made out of PDMS shows good replication of single cells and root hair emergence (bottom right image) and fully grown root hairs (bottom left image)
We are exploring a range of cellulose based materials that will mimic both surface structure and material properties. We developed a system based on Carboxymethyl Cellulose (CMC) that visualizes the enzymatic degradation of cellulose chains. The halo in the dyed CMC film appears only when cellulase enzyme is added but not in the presence of buffer as shown in A in the figure below. Benedict test shows that this is the result of CMC degradation to mono or di saccharides, as shown in B.
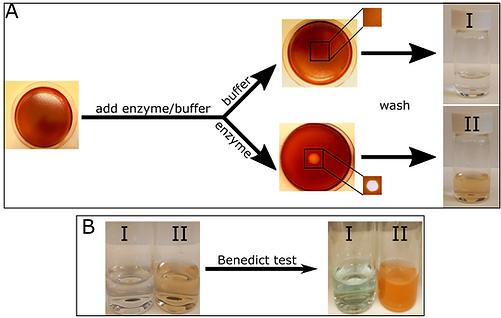
RKN invading a tomato root (from Wikipedia)
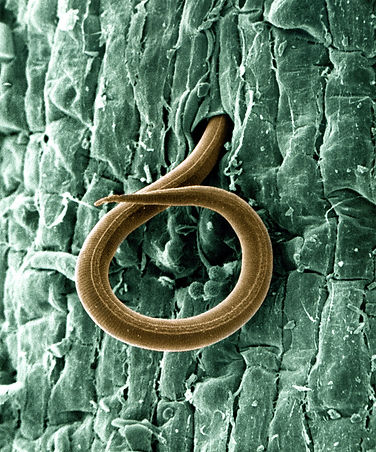
We are aiming towards a three-dimensional single root replica followed by a root system replica. The synthetic roots will be used as a model system to study the interaction with soil and different microorganisms, both beneficial and pathogenic. We are interested in structural features aiding in nutrient transfer, for example, as well as structural features necessary for pathogen resistance. One interaction we are interested in is between tomato root and the Root Knot Nematode (RKN) - a parasitic nematode attacking the plant through the roots and destroying crops.

The CMC system we built, capable of detecting cellulase activity is also reacting to RKN cellulase secretion, as can be seen in the small halos that appear in the CMC film marked by blue arrows. This happens at both 37°C and 27°C, though the reaction is less efficient at 27°C. Nematodes are clearly visible within halos. The reaction is amplified when root extract is added as seen by the large halo formed with root extract where nematode location is marked with green arrows.
3. The Effect of Platform Physical Properties on Callus Transition from Proliferation to Differentiation
Callus is a group of undifferentiated cells. These cells are known as proliferating cells, yet the right hormonal trigger can switch them into differentiating cells. We are testing the hypothesis that the physical properties of the platform the callus is cultured upon can also act as a trigger for this switch. Our preliminary data shows that in less compressible platforms, the callus tends to be more compact and shows more differentiation.


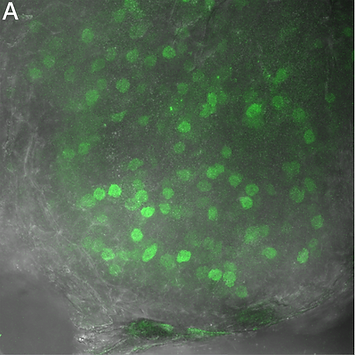
We are using transgenic plants with markers for cell proliferation, hormonal response and cytoskeleton - to monitor directionality of cell division. The image shows a callus from CYCB-GFP transgenic plant showing fluorescence only in replicating cells. This tool will assist in understanding the exact locations of proliferation within the callus.

We are designing different structures which we 3D print (A) and use as molds to pattern the platform (B). These structures will assist us in understanding whether the platform microstructure is also an important parameter for callus development.